The Holographic Metasurface Nano-Lithography (HMNL) process developed by my lab uses sub-wavelength-patterned metasurface masks (metamasks) to create multi-colored holograms in photocurable metal-polymer hybrid resins. This process allows entire 3D, multi-material (insulators and conductors) nanostructures to be patterned using a single light exposure. Metasurfaces can be encoded with much higher information density than traditional spatial light modulators such as digital micromirror devices, so the use of metasurface optics in HMNL overcomes many of the low-resolution and small-patterning-area challenges presented by conventional holographic 3D printing approaches. Additionally, the size of the hologram produced is limited only by the size of the fabricated metasurface. Using this method, we have demonstrated the ability to fabricate structures with ~500 nm resolution over areas greater than 30 mm × 30 mm. We have also demonstrated that metasurface optics can be designed to produce different holographic patterns when exposed to different colors of light and have developed a new resin chemistry that produces conductive metal structures when exposed to UV light and dielectric polymer insulators when exposed to visible light. By combining these two advances, we can build 3D metal-insulator composite structures at a rate of over 20 mm3/s.
You are here
Projects
Current Projects
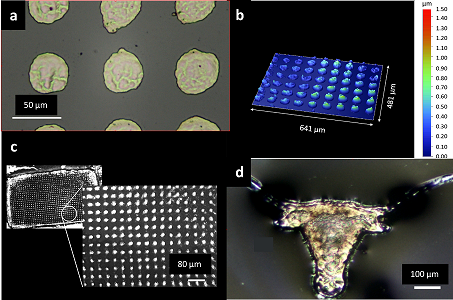
Additive manufacturing is well suited to making complex structures, but unfortunately current commercially available metal additive-manufacturing tools have feature-size resolutions of > 100 μm, which is too large for microelectronic interconnects. Research-grade metal additive manufacturing tools do exist, but their build rates are generally too slow for commercial applications. Therefore, the goal of the additive manufacturing work at the NDML is to improve the minimum feature-size resolution of metal additively-manufactured parts by up to two orders-of-magnitude, while still maintaining the throughput of traditional additive manufacturing processes. In order to achieve this goal, the NDML has developed a new microscale selective laser sintering system (μ-SLS) that uses micromirrors, high-speed nanopositioning stages, and a complex optical design to achieve write speeds on the order of 300 mm/s, and a feature-size resolution of ~ 1 μm. In this system, a laser is coupled to a fiber optic lens and directed off a micro-mirror array through a set of focusing objectives. This allows each 7.6 μm by 7.6 μm pixel in the micromirror array to be focused down to a spot size of approximately 1 μm. The μ-SLS also replaces the microscale powders used in conventional SLS processes with a nanoparticle (NP) ink. The use of NP ink in the μ-SLS system is required to build layers that are approximately 1 μm thick, because it is necessary to use particles that are at least one order-of-...
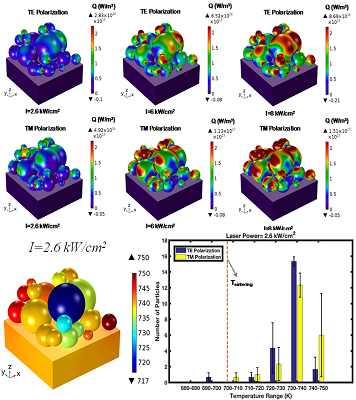
In addition to the experimental μ-SLS testbed itself, we have developed new NP-scale models of the μ-SLS build process. Modeling the μ-SLS process is challenging because most macroscale models of the SLS process rely on assumptions that are no longer valid when the size of the particles involved is smaller than the wavelength of the laser being used to sinter them. In modeling the μ-SLS process we must account both for the wave nature of light and the fact that the heat transfer in the μ-SLS process is dominated by particle-to-particle contact resistances, which change significantly as the NPs start to sinter together. Finally, the agglomeration of nano-particles in the powder bed must be accurately modeled in order to precisely predict the formation of defects in the final parts produced. The discrete element simulations coupled with finite element heat transfer models of the laser interaction with the NPs are used to determine how heat is generated within the NP bed and how that heat causes a rise in the bed temperature. Phase-field modelling is then used to predict how the nanoparticles in the μ-SLS powder bed will fuse together during the sintering operation. This modeling involves simulations of the laser-nanoparticle interactions,...

One type of device that is of particular interest to the NDML is graphene-based nanoelectromechanical resonators (GNEMS). This is because GNEMS can be used as both extremely sensitive mass/force detectors for biosensing applications and as highly tunable, reconfigurable electromechanical filters for communications applications. GNEMS resonators offer the potential to overcome many of the limitations of traditional MEMS-based resonators because of graphene’s outstanding electrical and mechanical properties. For example, graphene has an extremely high stiffness-to-weight ratio, which makes it possible to construct graphene resonators with very high natural frequencies. Also, graphene has a very high yield strain, which allows it to be stretched to very large tensions before failure. These properties allow us to tune the resonance frequency of a single graphene resonator over an extremely wide range (~ three orders of magnitude from MHz to GHz), which is important in communications and sensing applications.
One of the major challenges in producing highly accurate graphene-based sensors and filters is the poor fabrication repeatability of graphene resonators due to small variations in the residual stress and initial tension of the graphene film. This has meant that graphene-based nanoelectromechanical resonators tend to have large variations in natural frequency and quality factor from device to device. This poor repeatability makes...
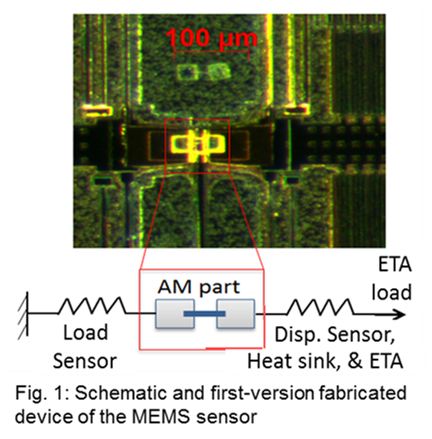
New polymer-based additive manufacturing (AM) systems have recently been developed that use 2-photon lithography to write 3-dimensional features with a resolution of approximately 200 nm. However, little is known about how processing parameters or the uncured polymer formulation effect the mechanical properties of the printed nanoscale structures. Therefore, we have developed a MEMS-based mechanical testing system onto which nano-AM structures can be printed in order to directly measure the mechanical properties of the 3D printed polymer structures. In this system, the nano-AM specimen is printed so that it is suspended between two MEMS stages. Each MEMS stage is connected to a set of capacitive sensors in order to be able to measure the displacement of each stage during testing. A thermal actuator is used to pull on one of the stages and to apply strain to the printed polymer sample. A capacitive sensor attached to the second stage is used to measure the total force applied to the sample. For precise and accurate characterization of a variety of print parameters, two different tensile testers have been created, one with a fine displacement resolution of 0.25 nm and the other with a high load capacity of 25 mN. Thus, this tensile tester can be used to generate the force-displacement curves for a wide verity of printed features, thereby enabling the characterization of the mechanical properties of nano-AM structures with unprecedented precision. This is a critical advance in our...
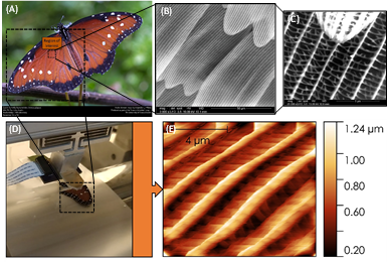
Through the application of compact, MEMS-based single chip atomic force microscopes (sc-AFMs) with high scanning speeds, we have developed a R2R metrology tool that greatly increases the throughput and modularity of direct, nanometer-scale measurement on flexible substrates. Designed to provide feedback for a R2R nanopatterning applications, the proof-of-concept system consists of a simple three roller unwind-idler-rewind web-handling arrangement, with the idler roller supported by radial air bearings to enable sensitive, on-roller measurements of the polycarbonate web. Two voice-coil driven vertical, double parallelogram flexure mechanisms are used to precisely position a gantry that holds the sc-AFM probe over the idler roller for web inspection. The nanopositioning sub-system quickly brings the sc-AFM probe within its 20 µm scanning height envelope on startup and rejects any external disturbances that could cause destructive tip-web contact. The probe is held at ideal scanning height for in-line measurement by compensating for idler roller eccentricity and topology using an offline map. Overall, this R2R nanometrology concept is highly scalable due to the modular nature of the fine-approach mechanism, sc-AFM sub-system, and parallelized, discrete control of each individual sc-AFM. We have also recently demonstrated that this system is capable of achieving sub-1 nm vertical resolution, 16.6 nm lateral resolution, and a throughput of 400 μm2/min with a web speed up to 2...
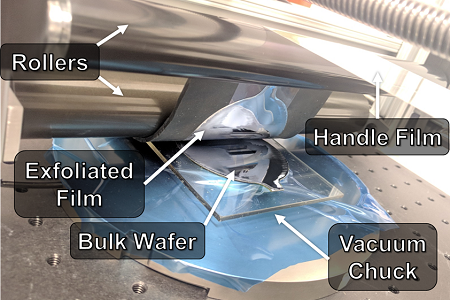
Single crystal silicon thin-films are extremely useful in a variety of applications where a combination of flexibility and high electronic performance is desired. The silicon exfoliation process is very cost-effective in creating thin-film Si compared with alternative methods such as etching or mechanical grinding. In the silicon exfoliation process nanoelectronic devices are first fabricated on a standard silicon wafer. A nitride passivation layer is then grown on top of the nanoelectronic devices, and a nickel layer is electroplated on top of the nitride in order to apply compressive stress to the silicon. This stress initiates a crack approximately 10 µm below the top surface of the silicon wafer, which can then be propagated along the wafer to produce a uniform, 10 µm thick, extremely flexible, single-crystal silicon film. To better control the exfoliation process, we developed an FEA-based fracture mechanics model which showed that by changing the angle of the applied load we could skew the mode mix ratio and, therefore, change the characteristic crack depth. Using this model, we designed a repeatable peeling tool that is capable of applying a constant exfoliation angle and force to the wafer during exfoliation. The tool features a simple, adjustable roller design attached to a linear actuator. The two-roller design moves a film over the wafer while maintaining a constant peeling angle and force. Testing has shown that by changing the tension in the...
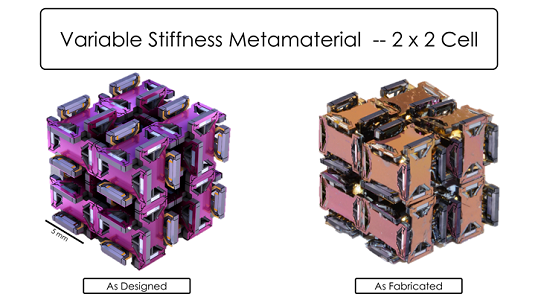
One new project in the NDML is the design, fabrication, and assembly of novel MEMS devices to create mechanical metamaterials with programmable stiffnesses. The MEMS devices used in this project are designed to have unique bidirectional thermal actuators which are capable of outputting large amounts of force in both tension and compression. The bidirectional MEMS actuators contain two actuating components, the inner actuator and outer actuator, both contained within an out-most enclosure. The outer actuator consists of a pair of multi-chevron beam structures mirrored and connected at the anchor and the inner actuator is a set of horizontal beams connecting the anchors through the handle layer. When a voltage is applied across the black electrodes, current flows through the outer actuator and the chevron beams undergo joule heating and thermal expansion. The outward horizontal motion at the anchors are restricted by the inner actuator beams, resulting in an upwards displacement at the tip of the device. Conversely, when a voltage is applied across the white electrodes, current flows through the center beams. The thermally expanded beams push the anchors outwards which results in a downwards displacement at the tip of the device. The MEMS devices also have piezoresistive strain sensors which are capable of very sensitive displacement measurements. Therefore, when the MEMS devices are assembled into a cube, they are capable of measuring an input force applied to the cube and then...
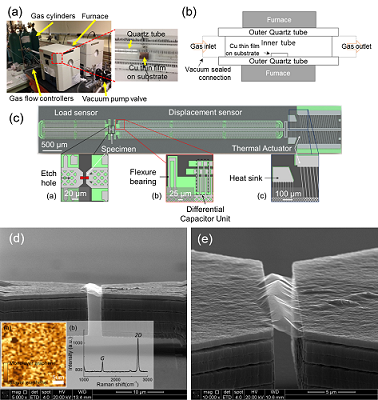
The goal of this research is to measure mechanical properties of graphene grown under different conditions which may affect the quality, grain sizes, and the number of layers of graphene. The mechanical properties of graphene are measured in many ways, yet conventional mechanical tensile testing of graphene sheets is difficult due to one atomic thickness of graphene and difficulties in handling graphene tensile specimen in nanoscale. We propose implementing Microelectromechanical System (MEMS) based tensile tester to accurately measure the mechanical properties of suspended graphene from strain versus stress curve.
Figures
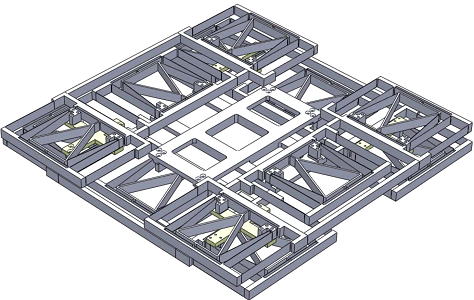
Long-range nanopositioning is a key enabling technology, catalyzing advances in a variety of existing and emerging domains such as scanning probe microscopy, semiconductor packaging and inspection, high-density data storage, micro additive manufacturing, and nano-metrology. Flexure bearings have emerged as a ubiquitous choice for nano-positioning applications since they experience low hysteresis and eliminate the effects of friction and backlash. The conflicting requirements of centimeter scale travel, high bandwidth, low stress concentration, and adequate safety factor make it challenging to design a compact flexure-based positioning stage. Existing designs demonstrate high accuracy and resolution, but acknowledge that large motion ranges are achieved at the cost of low resonant frequency.
The purpose of this research is to enable high-speed, long-range nanopositioning through computational optimization of Double Parallelogram (DP) flexure-based nanopositioning stages. The proposed methods can be used to design a platform with two degrees-of-freedom, meeting the required static and dynamic performance characteristics in a compact form-factor. By stacking planar DP units with optimized structural members, the proposed design can travel 50 mm in each axis with sub...
Past Projects
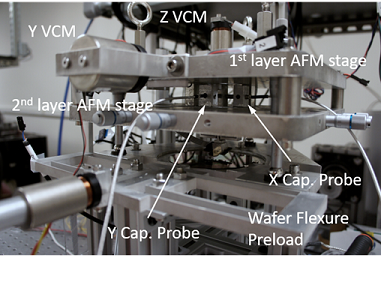
Atomic force microscopy is capable of producing very high resolution (sub-nm-scale) surface topology measurements and is widely utilized in scientific and industrial applications, but has not been implemented in-line with manufacturing systems, primarily because of the large setup time typically required to take an AFM measurement. In order to overcome this limitation, the NDML has developed a single-chip-AFM-based inspection system where a wafer can be precisely and repeatably loaded into the setup and measurements can be taken in under 60 seconds.
This inspection system consists of several single-chip AFMs integrated into a positioning stage to make measurements at multiple spots on a wafer at the same time, as shown in Figure 6. Each single-chip AFM is a MEMS device that is approximately 2 mm wide by 1 mm tall and is capable of scanning a 10 μm by 10 μm area. Thermal actuators in the MEMS device are used to do the scanning in both the x and y directions as well as to excite the z axis of the AFM so that it can be run in taping mode. Each AFM is attached to a flexure stage in the top plate of the inspection system so that the AFM can be precisely moved to the desired inspection location on the wafer. The flexure plate is coupled to the inspection plate using a kinematic coupling so that the flexure plate can be precisely located with respect to the inspection plate after each loading operation. In order to take a measurement, the...
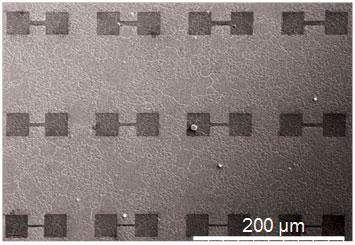
Most graphene-based NEMS devices are fabricated in a “one-off” manner using slow, limited scale methods such as mechanical exfoliation, electron beam lithography, or transfer from copper foils which can’t be incorporated into standard micro- and nanofabrication lines. In order to overcome this limitation, a method was developed that can be used to manufacture graphene-based NEMS devices at the wafer scale using conventional microfabrication techniques. In this project graphene is grown directly on thin film copper using chemical vapor deposition. The copper film is then patterned and etched to produce graphene-based NEMS resonators. This research is still in the early stages of development but this manufacturing method offers the potential to increase the throughput, yield, and repeatability of manufacturing graphene resonators while reducing the manufacturing cost and complexity.
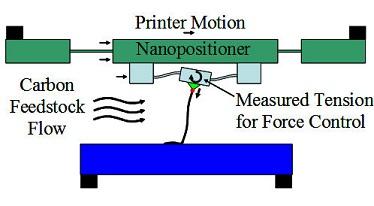
One of the greatest challenges in the development of nanoscale sensor systems and other nanomaterials-based devices is our inability to precisely, accurately, and repeatably place the desired nanomaterials and nanostructures where we want them, at a high rate and a low cost. For example, carbon nanotubes (CNTs) have long been seen as potentially transformative material due to their outstanding electrical (current density >109 A/cm2) and mechanical (elastic modulus > 1 TPa) properties. However, very few carbon nanotube-based devices exist due to our inability to manufacture CNTs at a large scale with the desired properties on any type of substrate. One potentially promising method for overcoming these is the direct printing of carbon nanotubes onto substrates. To achieve this type of direct printing, a chemical vapor deposition (CVD) furnace with integrated positioning and sensing to controllably grow and place CNTs onto a substrate is needed. This tip-based fabrication method would allow different types of nanotubes (single walled, multi-walled, functionalized, etc.) to be directly and precisely placed on prefabricated structures at a low cost and high speed.
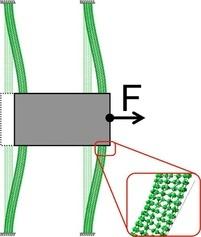
Carbon nanotubes (CNTs) may be used to create nanoscale compliant mechanisms that possess large ranges of motion relative to their device size. Many macroscale compliant mechanisms contain compliant elements that are subjected to fixed-clamped boundary conditions, indicating that they may be of value in nanoscale design. The combination of boundary conditions and large strains yield deformations at the tube ends and strain stiffening along the length of the tube, which are not observed in macroscale analogs. In this project molecular dynamics simulations are used to help model and design a nanoscale linear bearing system. These simulations show that the large-deflection behavior of a fixed-clamped CNT is not well-predicted by macroscale large-deflection beam bending models or truss models. However, pseudo-rigid-body modeling may be adapted to capture the strain stiffening behavior and, thereby, predict a CNT’s fixed-clamped behavior with less than 3% error from molecular simulations. The resulting pseudo-rigid-body model may be used to set initial design parameters for CNT-based compliant mechanisms. This removes the need for iterative, time-intensive molecular simulations during initial design phases.
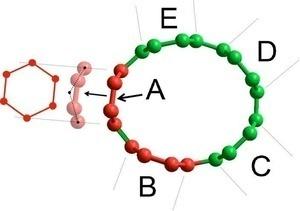
Molecular dynamics simulations can be used to examine the mechanical properties of carbon nanotubes when both axial and lateral forces are applied to the CNT. However, this project shows that an elastic tube model of a (5,5) carbon nanotube predicts stretching and bending moduli that differ by 19%. This is due to (1) differing energy storage mechanisms in each mode and (2) the inability of the tube model to capture these effects. Conventional tube models assume a common energy storage mechanism in stretching and bending. They show that energy is stored primarily through bond stretching/rotation and bond torsion/van der Waals interactions in stretching and bending, respectively. This knowledge underscores the need to use different moduli to predict stretching, bending, and combined bending and stretching when using the tube model.
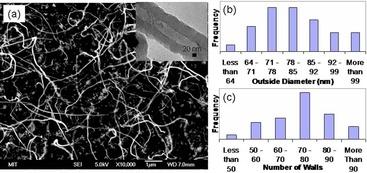
In this project a growth model was developed to control the diameter and number of walls in multi-walled carbon nanotubes grown by chemical vapor deposition (CVD). From this model, tunable process parameters may be used to control the geometry or stiffness of multi-walled carbon nanotubes (MWCNTs). This is important to devices that rely on the compliance of MWCNTs in order to achieve specific performance requirements, e.g. deflection or stiffness. Examples of these types of devices include relays, resonators and flexural bearings for small-scale actuators. It is necessary to control the stiffness of these mechanisms because the force, stroke, and device bandwidth depend upon the stiffness of the constituent MWCNTs. For a given length MWCNT, the stiffness is controlled by the MWCNT diameter and the number of walls in the MWCNT. The diameter and number of walls are controlled by adjusting several growth parameters – temperature, catalyst film thickness, and hydrocarbon concentration. Using thermodynamic relations, optimal growth parameters can be determined for CNTs with specific stiffnesses and natural frequencies. Overall, I was able to control the CNT geometry with less than 7 percent error and the stiffness and natural frequency with less than 2 percent error.
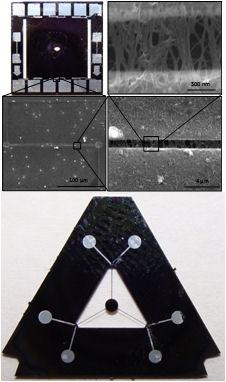
As mechanical devices move towards the nanoscale, smaller and more sensitive force and displacement sensors need to be developed. Currently, many biological, materials science, and nanomanufacturing applications could benefit from multi-axis micro- and nanoscale sensors with fine force and displacement resolutions. Unfortunately, such systems do not yet exist due to the limitations of traditional sensing techniques and fabrication procedures. Carbon nanotube-based (CNT) piezoresistive transducers offer the potential to overcome many of these limitations. Previous research has shown the potential for the use of CNTs in high resolution micro- and nanoscale sensing devices due to the high gauge factor and inherent size of CNTs. However, a better understanding of CNT-based piezoresistive sensors is needed in order to be able to design and engineer CNT-based sensor systems to take advantage of this potential. The purpose of this research is to take CNT-based strain sensors from the single element test structures that have been fabricated and turn them into precision sensor systems that can be used in micro- and nanoscale force and displacement transducers. In order to achieve this purpose and engineer high resolution CNT-based sensor systems, the design and manufacturing methods used to create CNT-based piezoresistive sensors were investigated. At the system level, a noise model was developed in order to be able to optimize the design of the sensor system. At the...
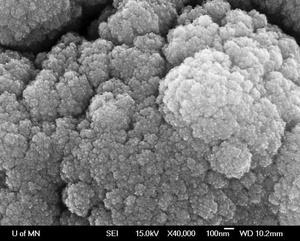
Si–Ti–N nanostructured coatings were synthesized by inertial impaction of nanoparticles using a process called hypersonic plasma particle deposition (HPPD). A study detailing the effect of plasma gases showed that in the case of an Ar+H2 plasma gas mixture, crystalline phases in the coatings consisted of TiN, TiSi2, and Ti5Si3. When the system was switched to a plasma gas mixture of Ar+N2, the only crystalline phase was TiN. Transmission electron microscopy confirmed the presence of TiN crystallites in an amorphous matrix. Warren–Averbach analysis indicated the average size of the TiN crystallites to be 14.9 nm. In separate experiments with the same conditions, aerodynamic lenses were used to deposit particles directly onto TEM grids. We observed agglomerated structures with an Ar+N2 plasma, while with an Ar+H2 plasma we found discrete TiSix and TiNx particles. In-situ particle diagnostics indicated only small changes in the particle size distributions when the plasma gases were changed.
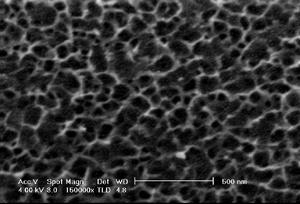
The excellent electrical and mechanical properties of porous nanostructured titania (NST) make it an ideal material for many different applications including dye sensitized solar cells (DSSC). While DSSCs are cheaper to manufacture than silicon-based cells, they are also less efficient. The efficiency of the DSSCs depends on how well the sensitizing dye coats the cell and how well the electron recombination source infiltrates into the porous NST. Consequently, the structure of the NST has a great effect on the efficiency of the cell. Different processing parameters were studied to determine their effects on pore size and surface area. Overall, it was found that increasing the deposition rate and the thickness of the original titanium film increased the pore size of the NST. Also, it was found that increasing the concentration of the hydrogen peroxide used to oxidize the titanium, increased the pore size of the NST. In general, it was also found that as pore size increased, surface area decreased.